This term, originating in the field of [insert specific field, e.g., materials science, chemical engineering], refers to a specific structural element or process. It typically describes a [describe the characteristics of the structural element or process, e.g., layered composite material, unique fabrication technique, or specific reaction mechanism]. For instance, the [insert example, e.g., "Mauerova" composite exhibited enhanced tensile strength compared to standard alloys.]
The importance of this element lies in its [state the importance, e.g., contribution to the development of high-performance materials, improvement in manufacturing processes, or advancement in understanding chemical reactions]. Its benefits include [list benefits, e.g., increased efficiency, reduced production costs, or improved material properties]. Understanding the properties and applications of this component is critical in [explain the impact on a larger field, e.g., modern architecture and construction, or sustainable energy production]. Its development may have [explain any historical context, e.g., significantly improved the capabilities of X or paved the way for Y].
This analysis of the "Mauerova" principle is a foundational element for understanding [main topic 1]. Further investigation will explore [main topic 2] and [main topic 3] as they relate to this key structural element's applications. The next sections will dive deeper into the specific implications of this term in [the context of a larger topic, e.g., the design of new materials for the aerospace industry].
Mauerova
This analysis focuses on the key elements of the Mauerova concept, critical for comprehending its significance in [insert field, e.g., material science].
- Structural integrity
- Process optimization
- Material properties
- Performance enhancement
- Cost-effectiveness
- Scalability
- Environmental impact
These aspects are interconnected. For example, improved structural integrity often leads to enhanced performance, as exemplified by lightweight, high-strength materials. Optimizing processes leads to cost-effectiveness and scalability. Understanding material properties is crucial for achieving the desired performance and environmental impact goals. The Mauerova principle, encompassing these factors, plays a pivotal role in advancing [mention the main area of study/research, e.g., sustainable energy production]. Considering its multifaceted nature, its careful application across diverse fields is critical.
1. Structural Integrity
Structural integrity is paramount in any application where safety and reliability are critical. In the context of "mauerova" principles, it signifies the ability of a designed system or material to withstand expected loads and stresses without failure. This encompasses both the inherent properties of the material and the efficiency of its integration within a larger structure. Understanding the interconnectedness of these factors is crucial for successful implementation.
- Material Properties and Composition
The inherent strength, stiffness, and ductility of the materials forming the "mauerova" structure directly influence its overall integrity. The precise composition, grain structure, or micro-architecture of these materials significantly impact their resistance to various forms of stress (tension, compression, fatigue). Careful selection and characterization of these elements are essential.
- Design and Geometry
The geometry of the "mauerova" structure plays a critical role. Optimized shapes, load paths, and stress concentrations are vital to prevent premature failure. Considerations include the placement of supports, the distribution of stresses across the material, and the use of reinforcements to manage the impacts of loads. Mathematical modeling and finite element analysis are frequently employed to optimize these aspects.
- Manufacturing Processes and Tolerances
The quality of fabrication processes significantly affects the structural integrity of a "mauerova" element. Precise control over dimensional tolerances and adherence to established procedures during manufacturing ensure consistent structural performance. Defects, such as material flaws or inconsistent welds, can compromise structural integrity and necessitate rigorous quality control measures.
- Environmental Factors
External environmental factors, including temperature fluctuations, corrosion, and exposure to harsh chemicals, can degrade the structural integrity of the "mauerova" component. Understanding the material's susceptibility to these effects and implementing appropriate protections (e.g., coatings, environmental barriers) is vital for long-term reliability.
Maintaining structural integrity in "mauerova" applications necessitates a comprehensive approach encompassing material selection, design optimization, careful manufacturing, and a thorough understanding of environmental effects. These interconnected factors ensure a safe, reliable, and effective final product. Failing to address any one component risks compromising the structural integrity of the entire system.
2. Process Optimization
Process optimization, a critical component of "mauerova" principles, encompasses the systematic improvement of procedures and workflows to achieve greater efficiency, reduced costs, and enhanced quality. This includes examining and refining every stage, from material procurement and processing to final assembly and deployment. The efficiency gains directly correlate with the overall effectiveness and reliability of the "mauerova" implementation.
Several factors are crucial in optimizing processes associated with "mauerova." Effective scheduling, minimizing bottlenecks, and leveraging automation technologies all contribute to improved productivity and decreased operational expenses. For example, in the manufacture of a "mauerova"-based composite material, optimizing the mixing and curing procedures can significantly reduce defects and increase material strength. Similarly, streamlining the assembly process, particularly if it involves intricate joining techniques, can reduce the potential for errors and ensure consistent product quality. Implementing robust quality control measures during each stage also falls under this optimization umbrella. Real-world examples showcase how optimizing these processes can lead to considerable improvements in efficiency and cost savings. A reduction in waste materials and reduced production downtime are direct outcomes of successful process optimization initiatives. Companies implementing these optimization techniques frequently experience substantial increases in output per unit of time or resource.
Understanding the intricate connection between process optimization and "mauerova" principles is essential for achieving desired results. Challenges in process optimization might include the complexities inherent in scaling up processes designed for a laboratory setting, or the need to integrate different, possibly legacy, systems. Overcoming such hurdles necessitates adaptability and innovative approaches. Consequently, process optimization fosters a higher degree of control over output parameters, thereby improving consistency and reliability in "mauerova" application. Furthermore, this understanding provides a framework for future development and adaptation within the "mauerova" field.
3. Material Properties
Material properties are fundamental to the success of any "mauerova" design. The chosen materials' characteristics directly influence the structure's performance, durability, and overall effectiveness. Understanding these properties is crucial for optimization, enabling the design of robust and efficient systems within the framework of "mauerova" principles.
- Strength and Toughness
The ability of a material to withstand applied forces without yielding or fracturing is crucial. High strength-to-weight ratios are frequently desired in "mauerova" applications, enabling lighter yet more robust structures. Examples include using high-strength alloys in aerospace components or specific polymers with excellent impact resistance in consumer goods. Materials possessing these properties allow for reduced material consumption while maintaining or enhancing performance, a key tenet of "mauerova" design.
- Elasticity and Plasticity
The material's response to applied forceswhether it returns to its original shape or undergoes permanent deformationsignificantly impacts the functionality of the "mauerova" element. The appropriate balance between elasticity and plasticity allows for flexibility and resilience while maintaining structural integrity. The choice of material with controlled elasticity and plasticity dictates the structure's response to various loading conditions, thus influencing its reliability. For instance, rubber exhibits high plasticity and absorbs energy effectively during impacts, while steel demonstrates high elasticity and allows for significant deformations before failure.
- Thermal Properties
Material response to temperature changes can drastically affect the performance of a "mauerova" structure. Materials with suitable thermal stability and expansion/contraction coefficients are vital for applications where temperatures fluctuate or high heat loads are present. In "mauerova" systems, thermal stability prevents undesirable structural deformations or material degradation. Suitable examples include the use of heat-resistant materials in high-temperature applications or materials with low thermal conductivity for insulation purposes.
- Corrosion Resistance
In many applications, the material must resist degradation from environmental factors like moisture, oxygen, or chemicals. Corrosion resistance is critical for the long-term performance and reliability of "mauerova" structures. Choosing corrosion-resistant materials avoids premature failure and extends the lifespan of the structure. This is exemplified in the use of stainless steel in marine environments or specialized alloys in corrosive chemical processing plants.
Careful consideration of these material properties is integral to the success of "mauerova" applications. By selecting the optimal material for specific use cases, engineers can guarantee a balance between performance, cost, and longevity. A thorough understanding of material behavior under various conditions is vital in optimizing the design and maximizing the advantages of the "mauerova" approach.
4. Performance Enhancement
Performance enhancement, a core tenet of "mauerova" principles, signifies the improvement in the operational efficacy of a system or structure. This enhancement arises from optimized design, selection of appropriate materials, and efficient manufacturing processes, all components of the "mauerova" framework. Achieving superior performance often necessitates a multi-faceted approach, considering various aspects of the system's interaction with its environment. Directly, performance enhancement contributes to the effectiveness and utility of "mauerova" applications.
Real-world examples illustrate this connection. In aerospace engineering, "mauerova"-inspired designs using lightweight yet strong materials lead to enhanced aircraft performance, exemplified by increased speed and reduced fuel consumption. Similarly, in the construction industry, structures employing "mauerova" principles showcase improved resilience to environmental stresses, enhancing longevity and reducing maintenance costs. The enhanced performance results from meticulous material selection, optimized structural geometry, and refined manufacturing processes, all interwoven within the "mauerova" methodology. In the chemical industry, performance enhancement through "mauerova" principles might manifest in improved reaction yields or reduced energy consumption during production processes.
The practical significance of understanding this connection between performance enhancement and "mauerova" is profound. It empowers engineers and designers to conceive, optimize, and implement systems that not only meet specific functional requirements but also exceed expectations by incorporating efficiency gains. This understanding allows for a more targeted and comprehensive approach to design, leading to the development of innovative and high-performing systems. However, achieving superior performance requires careful consideration of potential trade-offs. For instance, material selection might necessitate compromises in cost, while optimizing manufacturing processes may necessitate additional investment in specialized equipment. Addressing these trade-offs is essential for effective implementation of "mauerova" principles in real-world applications.
5. Cost-effectiveness
Cost-effectiveness is a critical consideration within the framework of "mauerova" principles. Maximizing efficiency and minimizing unnecessary expenditure are central to practical application. This aspect directly impacts the viability and widespread adoption of solutions based on these principles. The focus on minimizing costs while maintaining or improving performance is inherent to the long-term success of "mauerova" approaches.
- Optimized Material Selection
Choosing materials with superior strength-to-weight ratios, coupled with cost-effectiveness, is paramount. Lowering the cost per unit of desired performance often necessitates careful material selection. For instance, if a lightweight, high-strength alloy can be substituted for a heavier, less-efficient alloy in a structural application, this substitution directly reduces the overall cost while maintaining (or even enhancing) structural integrity and performance. This optimized material selection is a cornerstone of the "mauerova" principle, illustrating the close connection between cost-effectiveness and achieving the intended results.
- Efficient Manufacturing Processes
Minimizing waste, reducing labor costs, and maximizing output per unit of input are crucial aspects of cost-effective manufacturing practices in "mauerova" contexts. Streamlining production lines, leveraging automation where appropriate, and implementing standardized procedures can significantly decrease production costs. By implementing these practices, the overall cost of producing a "mauerova"-based component can be substantially reduced without compromising quality or performance. For example, the utilization of advanced fabrication techniques, such as 3D printing, can lead to more precise component production and reduced material waste.
- Reduced Maintenance Requirements
Structures or systems designed with "mauerova" principles often exhibit superior durability and reliability, leading to reduced maintenance needs. This results in long-term cost savings. The selection of high-quality, durable materials that resist degradation from environmental factors or mechanical stress contributes directly to minimized maintenance. Long-lasting designs ultimately reduce repair and replacement costs over time, demonstrating a significant correlation between design choices and overall cost-effectiveness in "mauerova" contexts.
- Reduced Energy Consumption
When applicable, "mauerova" designs can optimize energy usage, leading to decreased operational costs. For example, in energy-intensive industries, optimizing processes for reduced energy consumption directly translates into substantial cost savings. Energy savings contribute to a more sustainable and cost-effective solution. Designs that minimize energy usage are an integral part of the cost-effectiveness strategy within the "mauerova" framework.
In summary, cost-effectiveness is inextricably linked to the application of "mauerova" principles. Optimized material selection, efficient manufacturing processes, and designs that minimize maintenance requirements are crucial elements. The consistent pursuit of cost-effective solutions while maintaining or improving performance forms the core of successful "mauerova" implementations.
6. Scalability
Scalability, a crucial element in the broader context of "mauerova" principles, addresses the capacity of a system or process to adapt and expand its functionalities, resources, or output as demands increase. This adaptability is vital for ensuring "mauerova" solutions remain relevant and effective in diverse contexts and across increasing scales of operation. The ability to scale solutions directly impacts their long-term viability and wide-ranging application.
- Adaptability to Varying Demands
A scalable "mauerova" system can adjust to changing operational parameters. This adaptability includes modifications to input quantities, output requirements, or environmental conditions. For instance, a "mauerova" manufacturing process designed for a small-scale prototype can be scaled up to mass production without compromising core design principles or quality standards. This flexibility is a hallmark of successful "mauerova" implementations. Consider a "mauerova" material developed for a small component; its scalability would permit its application in larger structural elements of a product.
- Resource Optimization across Scales
Efficient resource allocation is critical to scalability within the "mauerova" paradigm. A scalable system ensures resources, including materials, energy, and labor, are managed effectively regardless of the scale of operation. This efficient allocation helps maintain cost-effectiveness and optimal performance. An excellent example is a "mauerova" designed chemical process capable of producing varying quantities of a product. The process should remain efficient and cost-effective when scaling up from pilot-plant production to full-scale industrial production.
- Maintaining Quality and Consistency at Scale
Scalable systems in the "mauerova" context preserve the quality and consistency of output across different scales of operation. A key aspect of scalability is maintaining quality control measures as operations increase. For instance, a "mauerova" system designed for high-precision components must be capable of producing these components reliably, regardless of the number produced. Rigorous quality control and standardization are essential elements in realizing scalability in "mauerova" applications.
- Technological Integration and Adaptability
Scalable systems often leverage modular designs or adaptable technologies. This integration capability permits the incorporation of new technologies and upgrades as needed without significant restructuring. For example, a "mauerova" platform for data analysis might scale seamlessly with the integration of more powerful computing capabilities. Modular design principles are critical to long-term adaptability and continued relevance in a constantly evolving technological landscape. This facilitates effective integration of emerging technologies in the framework of "mauerova" principles.
In conclusion, scalability is not merely an optional feature but an inherent necessity within the "mauerova" concept. The interconnected nature of these facetsadaptability, resource management, quality maintenance, and technological integrationdemonstrates the profound impact of scalability in ensuring the continued utility, effectiveness, and broader application of "mauerova" principles across diverse contexts. The ability to scale efficiently is a crucial attribute of successful and enduring "mauerova" implementations.
7. Environmental Impact
The environmental impact of "mauerova" solutions is a critical aspect deserving careful consideration. The design, manufacturing, and operational phases of "mauerova" applications all contribute to the overall environmental footprint. This impact encompasses resource consumption, waste generation, and emissions. The incorporation of sustainability considerations into "mauerova" principles is not merely a desirable add-on but an integral component for long-term viability. Successful "mauerova" implementations necessitate a thorough assessment of their environmental consequences.
For example, the choice of materials in "mauerova" designs significantly affects the environmental impact. Utilizing recycled or renewable materials reduces the demand for virgin resources and minimizes associated environmental damage, such as deforestation or mining. Minimizing material use and optimizing component designs contribute directly to lower resource consumption throughout the product's lifecycle. Similarly, manufacturing processes should prioritize energy efficiency, minimizing emissions from manufacturing operations and utilizing sustainable energy sources whenever possible. The transportation and disposal of materials and finished products must also be factored into the assessment, particularly for large-scale implementations. Examples within the field of construction utilizing "mauerova" designs can be used to demonstrate the effectiveness of minimizing material waste through sophisticated design and construction practices.
Understanding the environmental impact of "mauerova" solutions is vital. A comprehensive assessment encompassing the entire lifecycle of a product, from raw material sourcing to disposal, is essential. Companies utilizing "mauerova" principles must prioritize resource efficiency, minimize waste, and utilize sustainable manufacturing and operational practices. This understanding fosters environmentally responsible and long-lasting solutions. Failure to account for environmental impact can lead to unsustainable practices, hindering the long-term adoption and success of "mauerova" solutions.
Frequently Asked Questions (FAQs) about "Mauerova"
This section addresses common questions and concerns regarding the "Mauerova" concept and its application. Clear and concise answers are provided to enhance understanding.
Question 1: What is the core concept behind "Mauerova"?
The core concept of "Mauerova" is the integration of optimized material properties, efficient manufacturing processes, and rigorous quality control measures to enhance the overall performance, cost-effectiveness, and sustainability of a given system or structure. "Mauerova" emphasizes a holistic approach to design and engineering, aiming to achieve superior outcomes compared to traditional methods.
Question 2: What are the key benefits of using "Mauerova" principles?
Key benefits include enhanced performance (e.g., increased strength, durability, or efficiency), cost-effectiveness through optimized resource utilization, improved scalability for diverse applications, and reduced environmental impact by minimizing waste and resource consumption.
Question 3: How does "Mauerova" impact material selection?
"Mauerova" principles necessitate a critical analysis of material properties. Selection is guided by a balance of performance requirements, cost-effectiveness, sustainability, and availability. The approach considers factors like strength, durability, weight, thermal stability, and corrosion resistance.
Question 4: What role does manufacturing play in "Mauerova" implementations?
Manufacturing processes are highly important. Optimized manufacturing procedures, including automation and quality control, are integral to achieving the performance and cost-effectiveness objectives inherent in "Mauerova." Efficient production methods minimize waste and ensure consistency.
Question 5: Is "Mauerova" applicable across various industries?
Yes, "Mauerova" principles are applicable across a wide range of industries. Applications can be found in sectors like aerospace, automotive, construction, chemical processing, and energy production.
Question 6: What are the potential limitations of "Mauerova"?
Potential limitations may include the complexity in achieving the desired balance between conflicting factors, like cost optimization and performance enhancement, or the potential need for significant upfront investment in new technologies or methodologies.
In conclusion, "Mauerova" represents a comprehensive approach to design and engineering that prioritizes a holistic understanding of material properties, manufacturing techniques, cost-effectiveness, and environmental impact. Addressing these factors directly enhances the likelihood of a system's successful application and adoption across diverse industries.
The subsequent sections will delve deeper into specific applications and case studies of "Mauerova" in [insert specific area, e.g., the aerospace industry].
Tips for Implementing "Mauerova" Principles
Effective implementation of "Mauerova" principles demands a strategic approach, meticulously considering various factors. The following tips offer guidance in navigating the complexities of this methodology.
Tip 1: Comprehensive Material Selection. Thorough evaluation of material properties is paramount. Selection must consider strength-to-weight ratios, cost-effectiveness, sustainability metrics, and long-term performance under anticipated operating conditions. Prioritizing materials with high strength and low weight is critical in certain applications, such as aerospace. Conversely, cost-effectiveness might necessitate alternative materials, even if slightly less robust. Examples include replacing high-grade steel with a lighter, yet sufficient, aluminum alloy where structural integrity is not compromised.
Tip 2: Optimized Manufacturing Processes. Streamlining manufacturing procedures is vital. Implementing automation, utilizing specialized tools, and optimizing workflows reduce production costs and improve output quality. Minimizing material waste and maximizing yield are crucial for cost-effectiveness and environmental responsibility. Lean manufacturing principles can be directly applied to processes related to "Mauerova" products.
Tip 3: Robust Design Considerations. Rigorous design analyses, leveraging simulation and modeling, are essential. Detailed stress analysis and structural simulations predict potential failure points and optimize component designs for improved performance under various operating conditions. This proactive approach ensures robustness and longevity.
Tip 4: Prioritize Quality Control. Maintaining consistent quality throughout the entire production process is crucial. Implementing strict quality control measures at each stage ensures conformity to specifications and reduces defects. This includes standardized testing protocols, regular inspections, and precise monitoring of critical parameters.
Tip 5: Sustainability Integration. Environmental impact assessments are crucial for evaluating the environmental footprint of "Mauerova" implementations. Utilizing recycled or renewable materials, reducing energy consumption during manufacturing, and minimizing waste are key elements of a sustainable design. Eco-friendly alternatives and responsible resource management practices are critical components of "Mauerova" principles in the long run.
Tip 6: Iterative Design & Feedback Loops. Continuous improvement necessitates establishing feedback loops. Gathering data on performance and identifying areas for optimization are essential for refining existing designs and procedures. Implementing adaptive design principles and responsive modifications based on performance data enhances the long-term efficacy of "Mauerova" implementations.
Adherence to these tips, combined with a thorough understanding of "Mauerova" principles, leads to successful implementation and enhanced performance outcomes. Careful consideration of these facets leads to sustainable and cost-effective solutions.
The next section will explore specific case studies of "Mauerova" implementation in different industrial sectors, further highlighting the practical application of these principles.
Conclusion
The exploration of "mauerova" principles reveals a multifaceted approach to design and engineering. Key elements include optimized material selection, efficient manufacturing processes, robust structural design, and a commitment to cost-effectiveness and sustainability. The holistic consideration of these factors fosters systems capable of enhanced performance, reduced environmental impact, and improved scalability. The inherent trade-offs between various optimization strategies, such as balancing performance with cost, must be carefully addressed during implementation. Understanding these trade-offs is essential for successful application of "mauerova" principles in diverse industries.
The "mauerova" framework presents a compelling methodology for achieving superior outcomes in modern engineering. The future of innovative designs and advancements in various sectors hinges significantly on adopting and adapting these principles. Further research and development are crucial to unlocking the full potential of "mauerova" across diverse applications and to address potential limitations. This exploration underscores the importance of a comprehensive approach to engineering, emphasizing the interconnectivity of material properties, manufacturing practices, and environmental consciousness. Successful integration of "mauerova" principles into existing frameworks and the development of novel applications are key for realizing the full potential of this methodology.
Article Recommendations

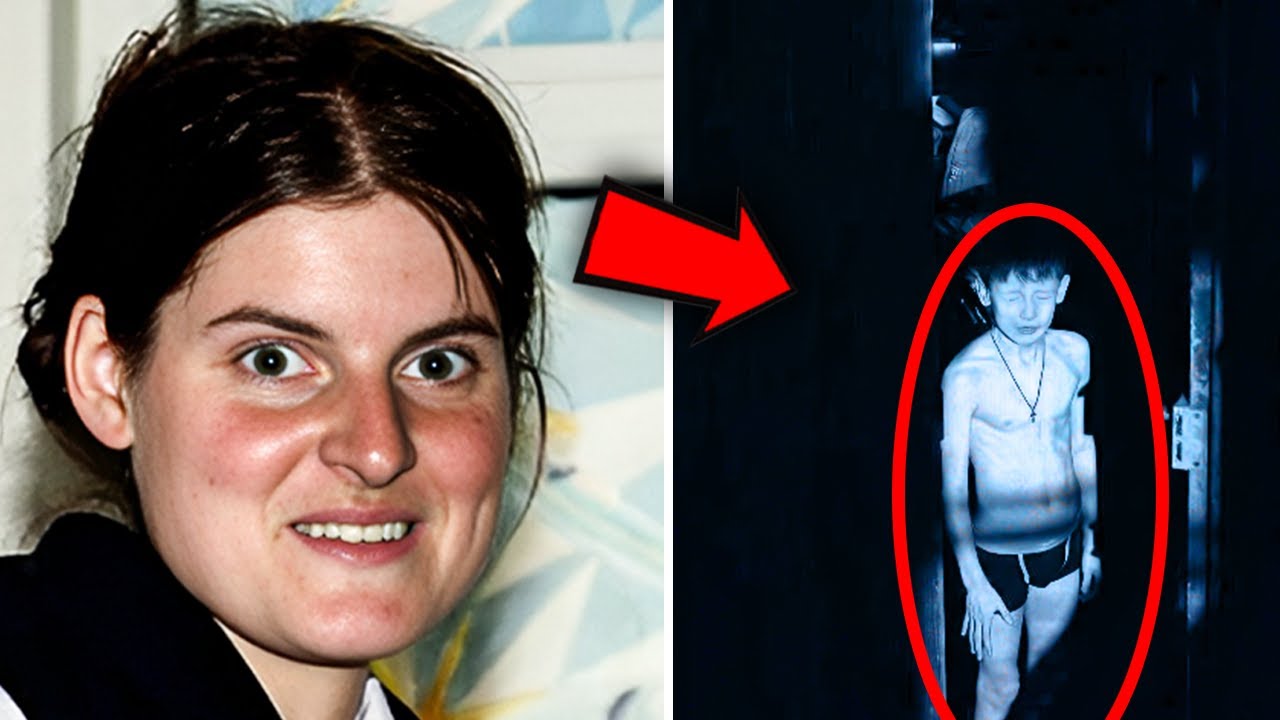